Rotator cuff tears are among the most common orthopedic injuries among adults in the United States, due to wear and tear or the effects of age. With a 94 percent failure rate for surgical repairs of large tears in older patients, it’s no surprise that the injury is a major cause of pain and disability.
With a five-year, $3.1 million grant from the National Institutes of Health, orthopedic researchers and engineers at Washington University in St. Louis are working to discover a better way to improve the outcome of surgical repairs by studying the natural attachment of tendon to bone. This understanding could lead to the engineering of new tissues that could enhance cuff repair.
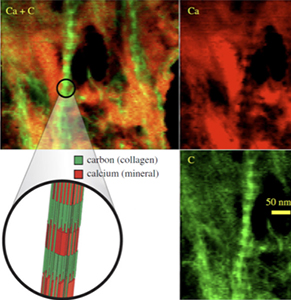
“Every motion you make is related to the attachment of tendon to bone,” says Guy Genin, PhD, professor of mechanical engineering in the School of Engineering & Applied Science. “The muscle is attached to the tendon, and the tendon is attached to the bone. Any break in the linkage will prevent motion, so this attachment is vital to the way the body works.”
The rotator cuff is a group of four tendons and muscles that connect the large arm bone to the shoulder. Surgeons repair large tears in the rotator cuff by removing any transitional tissue left behind at the injury site and suturing the tendon directly to the bone.
“The natural attachment system is not regenerated during healing, even following surgical repair,” says Stavros Thomopoulous, PhD, associate professor of orthopaedic surgery in the School of Medicine. “The healing process leads to scar tissue formation at the healing tendon-to-bone interface, and the resulting attachment is prone to re-injury.”
Thomopoulous and Genin are leading an interdisciplinary and multi-institutional group of researchers to reverse-engineer the tendon-to-bone attachment, looking at the toughness and resilience of the tissues to better understand how the natural tendon-to-bone insertion works and what goes wrong in healing.
“When material is structured like tendon and bone, mechanisms for smooth attachment and transfer of stress are harder to design,” Genin says. “Nature has a great design, and surgical repair techniques might be improved by better understanding this. We’re applying our experience in bridging length scales and modeling toughness and resilience to tissues to try to understand both how the natural tendon to bone insertion works and what goes wrong in healing.
“We need engineering approaches to determine what’s important,” Genin says. “What needs to be recreated in healing, and what is an artifact of development that brought it to that point?” Genin says.
The team plans to study the tendon-bone attachment using a variety of imaging methods working with Mark Anastasio, PhD, interim chair and professor of biomedical engineering in the School of Engineering & Applied Science. They will use scanning transmission electron microscopy-electron energy loss spectroscopy to determine mineral and collagen distributions at the site of insertion of tendon to bone and perform mechanical testing on the collagen fibers. The work will be the first mechanical measurements of collagen fibers derived from mammals and the first validation of associated molecular dynamics calculations.
In addition, they will use synchrotron X-ray diffraction, Raman spectroscopy and polarized light microscopy to determine the distributions of mineral content and collagen orientation along the tendon-to-bone insertion. They will also use phase contrast X-ray computer tomography to determine the 3-D geometry of tendon and bone and tissue-level testing to determine the mechanics of the tendon-to-bone insertion.
Additional WUSTL collaborators include Leesa Galatz, MD, associate professor of orthopaedic surgery who specializes in rotator cuff repair, and Jill Pasteris, PhD, professor of earth & planetary sciences and a mineralogist with expertise in bone mineral. Scientists at the Massachusetts Institute of Technology, University of Illinois and University of Pennsylvania complete the team with wide-ranging expertise in modeling and experimentation.
“Results from mechanical testing, imaging and spectroscopy studies will allow us to develop new multiscale models to describe the mechanics of the tendon-to-bone attachment,” Thomopoulos says. “These modeling tools will allow tissue engineers to develop novel scaffolds for tendon-to-bone repair. Understanding this complex natural system may also inspire new approaches for attaching materials in structural engineering.”
Source: Washington University in St. Louis
Was this article valuable?
Here are more articles you may enjoy.